Polymyxin B is a last-resort antibiotic used to treat many hospital-acquired bacterial infections. It belongs to a class of lipopeptide antibiotics that combat multidrug-resistant Gram-negative infections caused by microbes like Klebsiella pneumoniae and Pseudomonas aeruginosa.
Understanding the interactions of such antibiotics with the OM of Gram-negative bacteria is of vital importance because four out of six ESKAPE organisms responsible for the majority of hospital acquired infections are Gram-negatives. These hardy bacteria are responsible for life-threatening diseases like pneumonia, sepsis and meningitis, making them key targets for clinical research. The more we can learn about these bacterial membranes and the interactions of drugs with them, the greater chance we have of targeting the looming threat of antibiotic resistance and the serious consequences this will have for our ability to treat disease.
In a world-first, the team were able to use an artificial model of the OM to not only explain why PmB only works at or above human body temperature but also support the notion that bacteria actively control the fluidity of their OM's as growth temperatures vary. The OM model – originally described in 2013 and analysed using state of the art neutron technology at the ISIS Neutron and Muon Source – provides a realistic framework that accurately reproduces the characteristic asymmetric structure and impermeability of the Gram-negative OM.
Polymyxin B was the antibiotic of choice for this experiment because it has an unusual and distinctive way of interacting with the membrane which makes it invaluable for the future design of novel antimicrobials.
How does antibiotic resistance develop?
Antibiotics are drugs that are used to kill bacteria or halt their reproduction and dissemination. They have saved millions of lives since their serendipitous discovery back in 1928. In fact, it is estimated that the very antibiotic that Fleming stumbled across in his lab, Penicillin, has saved between 80 and 200 million lives worldwide.
Through natural means, bacteria, like any other organism, evolve with time and are subject to the forces of natural selection. Picture a scenario like this. Within a bacterial population you have a large amount of genetic variety. Perhaps within this population, at least one bacterium has undergone a genetic mutation that has bestowed upon it resistance to an antibiotic – let's say tetracycline. In the absence of tetracycline, this bacterium does not pose a threat and will remain a rare example. However, if the individual who houses this bacterial population starts a treatment of tetracycline antibiotic, maybe to treat a urinary tract infection, the scenario changes. Bacteria with resistance have what biologists call a 'selective advantage' over other bacteria and can survive in the presence of the antibiotic treatment. As their bacterial peers are killed off, the resistant bugs survive to reproduce and spread. If these bacteria acquire new antibiotic resistance genes from other species, superbugs result, that is, bacteria that are resistant to more than one type of antibiotic. In 2017, for example, public health officials in Nevada reported that a woman had died from an 'incurable infection.' Tests revealed the presence of a Klebsiella pneumoniae superbug that was resistant to 26 different antibiotics.
The more we use antibiotics, the more we speed this natural process up. Whilst Public Health agencies campaign to cut down their use, scientists from all over the world are working hard to find new antibiotics or to redevelop existing ones.
How can technology developed at ISIS Neutron and Muon Source help?
In October 2015, Professor Jeremy Lakey from Newcastle University's Medical School and Dr Luke Clifton from STFC's ISIS made news for their paper chronicling a world-first; the creation of a working model of the OM of Escherichia coli, a bacterium that is notoriously hard to study in vivo owing to its size and the complexity of its cell wall. Now they are using this model to specifically investigate the molecular mechanisms by which one particularly important antibiotic interacts with the membrane of Gram-negative bacteria.
The antibiotic in question, Polymyxin B, is a mixture of closely related LPS-binding amphiphiles derived from the bacterium Bacillus polymyxa. Its status as a last resort treatment against otherwise-resistant Gram-negative infections means that it is a hot topic in microbiology, especially with regard to the unique manner in which it interacts with the OM.
The way PmB works is by disrupting the OM structure, which leads to the loss of lipid asymmetry and an increase in leakiness leading to cell death. This contrasts with most of the antibiotics used to kill Gram-negatives, such as Beta lactams, which avoid the hydrophobic core of the OM, entering the bacteria instead via water-filled protein pores that are normally used for the uptake of soluble nutrients. Whilst these antibiotics have been very successful in recent years, the pore proteins are evolving to limit their permeability and, when combined with other resistance mechanisms, this seriously reduces drug efficacy.
To solve this problem we need a better understanding of the properties of the OM and how antibiotics interact with it.
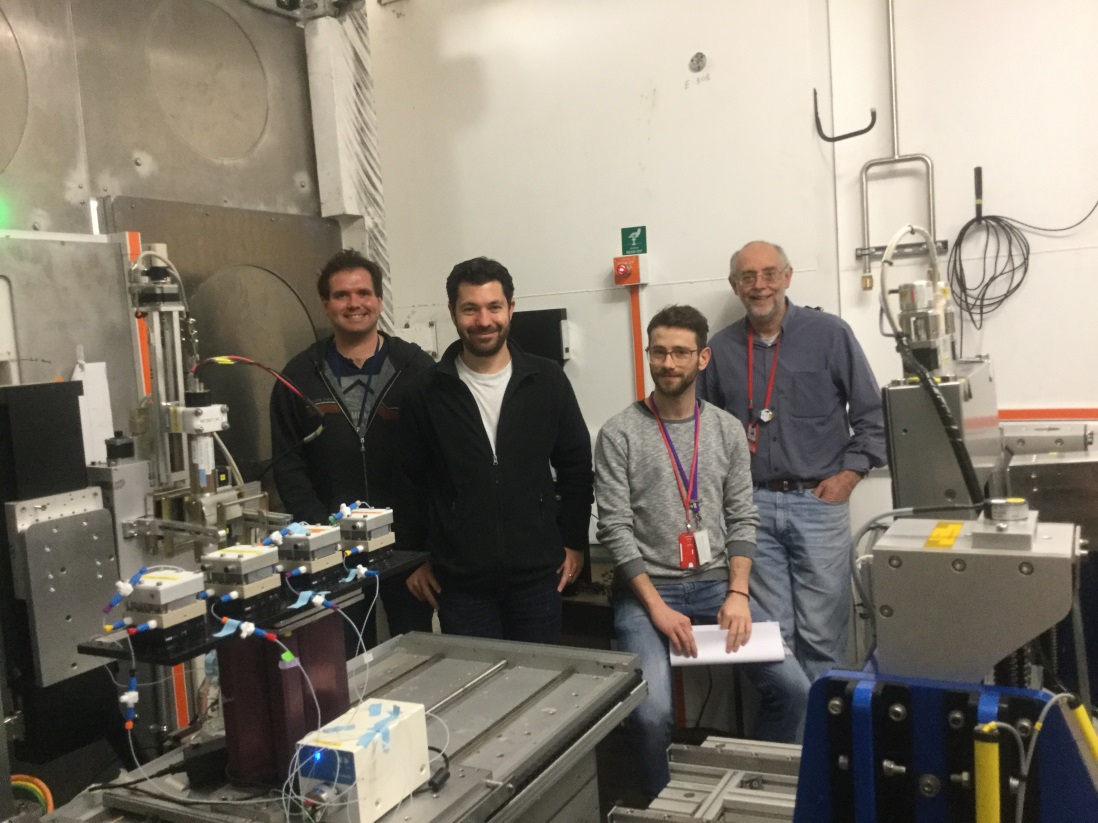
From left to right we have: Luke Clifton, Maximilian Skoda, Nicolo Paracini and Jeremy Lakey next to POLREF.
The future
For many years, the OM of Gram-negative bacteria, which is 25,000 times thinner than a sheet of A4 paper, has been very difficult to characterize in vivo. The creation of a working artificial Gram-negative bacterial membrane at ISIS therefore provides a concrete method for delving deeper into the chemical specifics of drug-membrane interactions.
Neutron reflectivity using the INTER reflectometer at ISIS and infrared spectroscopy allowed the scientists to study both leaflets of the bacteria at the same time, providing unprecedented insight into the physico-chemical properties of the antibacterial compound.
Based at the Newcastle University's Centre for Bacterial Cell Biology and ISIS, the interdisciplinary collaborative team are already working on new models which incorporate more complex lipids and proteins to more closely imitate the living bacteria and allow studies of a wider range of antimicrobials.
The results, published in the prestigious PNAS (Proceedings of the National Academy of Sciences of the United States of America) journal, show how the physical state of the lipid matrix of the OM is a critical factor in regulating the interaction with the antimicrobial peptide. Initially the team were surprised that different models of the OM of Gram-negative bacteria produced different responses to PmB. It then became clear that such differences were due to subtle differences in the molecular packing. Only tightly packed OM models at body temperature imitated the clinical conditions; at room temperature the model was frozen and would not interact with the antibiotic. Thus PmB relies upon microscopic movements of the membrane molecules to slip in and disrupt the OM structure.
“The outer membrane of the Gram negative bacteria is the barrier between these microorganisms and their external environment. The models of this surface we have developed with our collaborators at Newcastle University allow us to gain molecular level details on this surface under conditions which match those found in the living organism. Neutron beam science is key to these studies as neutrons are a highly adaptable, non-damaging probe of molecular structure which can differentiate between the differing biomolecules found in these complex systems. This allows us to locate the individual components within the bio-membrane and examine how these components change during the interaction of the antibiotic"
Dr Luke Clifton, ISIS Neutron & Muon Source
“At Newcastle University we have a large number of research groups developing new antibacterial treatments. In my group we look for weaknesses in the bacterial defences against antibiotics. This paper shows how we can use our neutron science to understand how bacterial defences work. In essence we now know that in order to live the bacteria need their defensive walls to be flexible but that this flexibility renders them susceptible to antibiotic attack. It's just the type of Achilles' Heel we are looking for."
Professor Jeremy Lakey, Newcastle University
"To face the rising threat posed by antibiotic resistant bacteria, we must have a detailed understanding of the mode of action of current drugs in order to improve their efficacy. Polymyxins are particularly relevant antibiotics because they are the last resort treatment for resistant Gram-negative infections and display an unusual mechanism of action. The molecular details of the activity of polymyxin B presented in this paper will aid the development of new antibiotics that are needed to face the ongoing antibiotic resistance crisis."
Nicolo Paracini, Newcastle University
Further information
The research was supported by STFC, Newcastle University and OJ-Bio Ld. The full publication is available to view in PNAS.
For further information about the research, please contact Dr Luke Clifton (ISIS Neutron and Muon Source) or Professor Jeremy Lakey (Newcastle)
To learn more about the antimicrobial research taking place at ISIS Neutron and Muon Source, please see this article.
Find out more about INTER.
Browse all our science highlights here.