As the population, and our individual energy needs, continues to surge there is a growing global demand for energy. To help solve this problem scientists have been developing a new source of energy that would provide us with safe, non-carbon emitting, and virtually limitless energy – fusion power.
We need look no further than the sun to see a shining example of fusion in action. In the extremes of pressure and temperature at the centre of the sun atoms travel fast enough to fuse together, releasing vast amounts of energy. This process has not gone unnoticed, for decades scientists have been attempting to bottle a mini star of our own right here on Earth.
Whilst we haven't achieved commercially viable fusion just yet, fusion energy projects around the world are bringing the dream of virtually limitless energy closer to reality. Dr Elizabeth Surrey of UKAEA explains one of the main approaches to fusion, magnetic confinement, which uses a tokomak design, “under the influence of tremendous heat and pressure, gaseous hydrogen atoms become a plasma—the very environment in which hydrogen atoms can fuse to yield energy. In a tokomak we use powerful magnetic fields to confine and control the plasma."
The components in fusion devices must cope with staggering temperatures, up to 10 times the heat of the centre of the sun. In light of this, researchers from the UK, Germany and France visited ISIS Neutron and Muon Source to image parts for use in a fusion energy device, pairing x-ray and neutron imaging for this purpose for the first time.
A component able to withstand 10 MWm-2
Magnetic confinement reactors have some of the greatest temperature gradients on earth, and potentially in the universe, with plasmas reaching highs of 150 million °C and the cryopump which is only meters away as low as -269 °C. As the gravitational forces present at the centre of the sun cannot be recreated on Earth, fusion energy devices must reach significantly higher temperatures to compensate.
Dr Llion Evans of Swansea University explains more, “a vital component of any tokamak is the divertor, which extracts heat and impurities from the plasma. Due to its location and function within a tokamak, the divertor is the component subjected to the greatest steady thermal load. To remain within operational temperature limits the divertor components are actively cooled. This is achieved by connecting armour tiles through their centres to a pipe carrying coolant, coined a monoblock.".
ITER, a magnetic confinement reactor under construction in France, will use monoblocks composed of a tungsten armour and copper alloy cooling pipe. The armour is bonded to the pipe to maintain thermal conduction, but a thermal expansion coefficient mismatch between the tungsten armour and copper alloy cooling pipe can cause high levels of stress within the part.
With 320,000 monoblocks required for ITER alone, it is of great importance for researchers to test the robustness of new, and pre-existing, engineering components that must function in such an extreme environment.
Imaging using complementary probes
In their latest research paper, scientists from Culham Centre for Fusion Energy, Swansea University, ITER, and the Max-Plank Institute of Plasma Physics paired x-ray and neutron imaging to study parts for use in a fusion energy device.
ISIS Neutron and Muon Source's neutron imaging instrument, IMAT, was used to gather information on these critical components. “Each technique had its own benefits and drawbacks. The advantage of neutron imaging over x-ray imaging is that neutrons are significantly more penetrating through tungsten. Thus, it is feasible to image samples containing larger volumes of tungsten. Neutron tomography also allows us to investigate the full monoblock non-destructively, removing the need to produce “region of interest" samples" adds Dr Triestino Minniti of STFC
In order to examine the structural integrity of the specimens two separate samples were tested in the experiments, one of which was as-manufactured, the other had been tested under high heat flux conditions.
Due to the differences in the neutron tomography technique, images will have a lower baseline resolution than what is possible with X-ray tomography. However, neutrons offer other advantages, they are more penetrating through tungsten and the full monoblock can be studied, rather than just a “region of interest" sample.
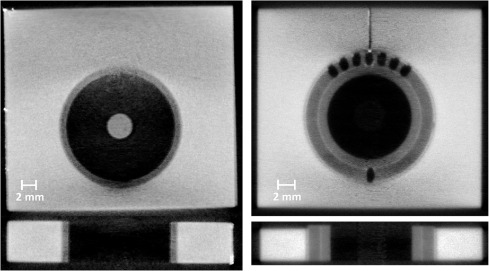
Above: example cross sectional tomography data slices from the xy (top) and xz (bottom) planes for the CCFE_MB_ROI sample with a comparison of X-ray (left) vs. neutron (right) tomography imaging results.
Below: example cross sectional neutron tomography data slices from the xy (top) and xz (bottom) planes for ITER_MB (left) and CCFE_MB (right) samples.
It is believed that this is the first time that a full tungsten divertor monoblock has been imaged by computerised tomography. The capability to inspect the material interfaces within the monoblock is of great value and the techniques shown in the paper show great promise. Dr Llion Evans concludes, “whilst insufficient samples were tested to draw conclusions on the candidate designs shown here this work is a proof of concept that both these tomography methods can produce valuable data. In future these complementary techniques can be used either for the research and development cycle of fusion component design or in quality assurance of manufacturing".
The next step is to convert the 3D images produced by this powerful technique into engineering simulations with micro-scale resolution. This technique, known as image-based finite element method (IBFEM), enables the performance of each part to be assessed individually and account for minor deviations from design caused by manufacturing processes.
The allure of nearly limitless clean energy has prompted billions of pounds of research, pushed engineering to the limit, and inspired scientists from around the world. This research demonstrates the small, but important, role neutrons may play in this challenging endeavour that's decades in the making.
Further information
The full research paper can be found here: Ll. M. Evans et al. “Comparison of X-ray and neutron tomographic imaging to qualify manufacturing of a fusion divertor tungsten monoblock" Fusion Engineering and Design 134 (2018) 97–108 98 DOI:10.1016/j.fusengdes.2018.06.017
Browse all our science highlights here.
Find further information on IMAT can be found on the webpage.
Further information on ITER can be found here.