The world is on the brink of climate catastrophe. In the current UN climate summit, COP27, it has been emphasised that the world is on the track for a temperature rise of 2.4°C by the end of the century. This rapid global warming is the result of using fossil fuels as a source of energy and so, to mitigate this challenge, renewable energy sources and storage devices have become a necessity. As renewable energy produced by solar and wind technologies is intermittent, there is a need for cheap storage devices that have long lifecycles.
Electrochemical batteries are a highly promising solution for this type of storage. Across ISIS, scientists are studying a range of battery materials and their properties, including conventional lithium-ion batteries and the next-generation of battery materials. As lithium (Li) is very expensive, and there are concerns over its scarcity and geological distribution, scientists are on the lookout for alternative materials. One such alternative is sodium (Na), because of its large global abundance.
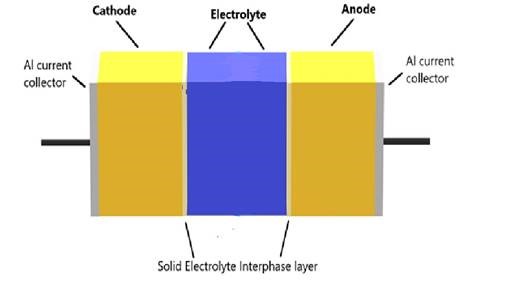
Solid-state batteries (as shown, right) are the most compact and safe version of electrochemical energy storage devices at various scales. These batteries contain a solid electrolyte rather than a liquid between the two electrode materials. This means no separator is required between electrodes as the solid electrolyte itself acts as a separator. As well as not needing a separator, solid electrolytes are more chemically stable than liquids, and less susceptible to leakage, evaporation, and flammability, which makes them safer.
Solid-state batteries also have a higher energy density and therefore have the potential to offer great advantages as batteries in electric vehicles. Also, metallic lithium or sodium can be used as an anode material in these batteries, increasing their current-carrying capacities.
Understanding the diffusion process of Li+ or Na+ ions in these electrolytes is important, as faster movement of these ions increases the battery's storage capacity. Quasi-elastic neutron spectroscopy (QENS) is a spectroscopic technique that is a great tool for investigating these diffusion processes, as the motions of particles can be explored at the atomic scale.
ISIS scientists, working in collaboration with Bhaba Atomic Research Centre, India, used QENS on the OSIRIS instrument, alongside ab-initio molecular dynamics (AIMD), to understand the Na- and Li-ion diffusion mechanism within solid-state electrolytes. They also used inelastic neutron scattering on MARI. In a series of recent studies, the group looked at a range of electrolyte materials to see how their structure influenced the ionic diffusion. For a battery material, high ionic diffusion is favourable for the charge/discharge process.
Their experiments were challenging, as both Li and Na have very small neutron cross sections, which makes data analysis difficult. Using AIMD alongside the experiments was extremely helpful in enabling them to interpret the data.
In the solid electrolyte material Li2Si2O5, they found that the dynamics of silicon polyhedral units plays an important part in the lithium diffusion process. In this case, the polyhedral units are rigid and provide only a narrow temperature window (between 700–775 K) where the fast motion of Li+ ions is observed. The researchers found that, when the lithium motion was fast, a superionic amorphous phase of Li2Si2O5 was present, making it likely to be responsible for these properties.
They saw similar behaviour in LiAlGeO4, where excess Li+ stoichiometry increases the Coulomb repulsion and softens the host structure of aluminium and germanium polyhedral units, AlO4 and GeO4, respectively, enabling faster Li+ ion diffusion along the hexagonal c-axis, as shown in the diagram below.
In amorphous Na2Si2O5, another solid-state electrolyte material, they found that the Na+ ions jump from one atomic site to the next, with an average jump length of about 3 Å, with a residence time about 9 ps. Their AIMD simulations revealed that in the amorphous phase there are many different orientations of the silicon polyhedral units, meaning that there are several pathways accessible for Na+ ion diffusion.
These pathways are absent in the crystalline phase due to rigid spatial arrangement of silicon polyhedral (SiO4) units, reducing the mobility of the ions.
On the other hand, in Na2Ti3O7, they found that the motion of Na+ is mostly localised and only one-dimensional diffusion is present, through an interstitial site, and only at high temperature, as shown in the diagram on the right.
These studies show that manipulating the ionic stoichiometry of these materials and prompting the formation of the amorphous phase can significantly improve the diffusion process, improving the material's potential performance in a battery. This is an important consideration to take into account when designing future solid-state battery materials.
The full papers can be found at:
https://pubs.acs.org/doi/10.1021/acsaem.2c02663
https://pubs.rsc.org/en/content/articlelanding/2023/TA/D2TA08170A
https://pubs.rsc.org/en/content/articlelanding/2022/MA/D1MA00963J
https://journals.aps.org/prmaterials/abstract/10.1103/PhysRevMaterials.4.045802