Fundamental structure of network glasses
E. R. Barney, A. C. Hannon, D. Holland, N. Umesaki, M. Tatsumisago, R. G. Orman and S. Feller, Terminal oxygens in amorphous TeO2, J. Phys. Chem. Lett., 2013, 4, 2312-2316.
O. L. G. Alderman, A. C. Hannon, D. Holland and N. Umesaki, On the germanium-oxygen coordination number in lead germanate glasses, J. Non-Cryst. Solids, 2014, 386, 56-60.
An understanding of the structure of a network glass starts with knowledge of the structural unit(s) on which the network is based, and this depends in turn on a knowledge of the coordination numbers and bond lengths. Accurate coordination numbers, however, are surprisingly difficult to measure for glassy materials, but the ability of GEM to measure these is unsurpassed by any other neutron diffractometer in the world, and is certainly better than can usually be achieved by X-ray techniques (diffraction or EXAFS). A good example concerns amorphous TeO2; there has been considerable interest in amorphous TeO2, because it is the base glass for many tellurite glass systems of technological interest, but for many years it was not clear whether or not the Te-O coordination number is four, as in related crystal structures. However, after careful investigation, GEM has shown the Te-O coordination number to be 3.68. This value differs significantly from four, showing that the glass structure contains a significant proportion of terminal oxygens, reminiscent of P2O5 glass. The result is consistent with Raman and NMR results, and leads to a structural model that explains a change in structure and properties that occurs at a composition of about 85 mol% TeO2 in binary tellurite glasses.
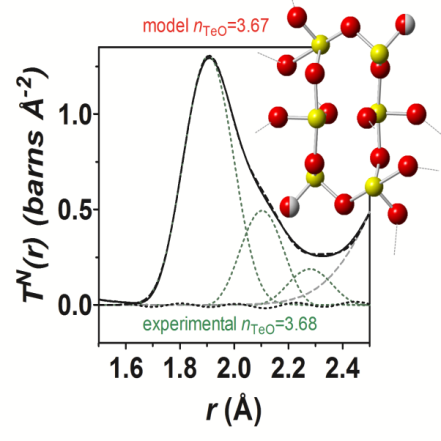
Neutron correlation function of amorphous TeO2 and illustrative network fragment for model with terminal oxygens (shown as two-tone spheres).
Bioactive glasses
U. Patel, K. M. Z. Hossain, A. R. Kennedy, E. R. Barney, I. Ahmed, R. M. Moss, and A. C. Hannon, Structural and physico-chemical analysis of calcium/strontium substituted, near-invert phosphate based glasses for biomedical applications, Acta Biomaterialia, 2017, in press.
Bioactive glasses are surface reactive glass-ceramic biomaterials, which are being investigated extensively for use as implant materials in the human body to repair and replace diseased or damaged bone. The technology is already applied in SensodyneÒ Repair & Protect toothpaste; this is a product of the British pharmaceutical company GlaxoSmithKline that uses NovaminÒ glass particles. The UK has a very strong scientific research effort in the area of bioactive glasses, with a large number of groups working in this field. There have been many GEM experiments on bioactive glasses, leading to the publication of ~30 papers so far. In this field, neutron diffraction is commonly used as one of a large number of experimental probes in a multi-technique study to characterise the bioactive glass. It is important to appreciate that there is a large body of work in this area, rather than to solely focus on one paper used as a highlight. For these studies, the high real-space resolution of GEM and the potential to measure accurate coordination numbers are vital for meaningful structural studies. This is illustrated well by the figure, showing data for MgP2O6 glass, where the high real-space resolution is important both for splitting the bonds from phosphorus to non-bridging and bridging oxygens, and for showing the asymmetric distribution of Mg-O bonds.
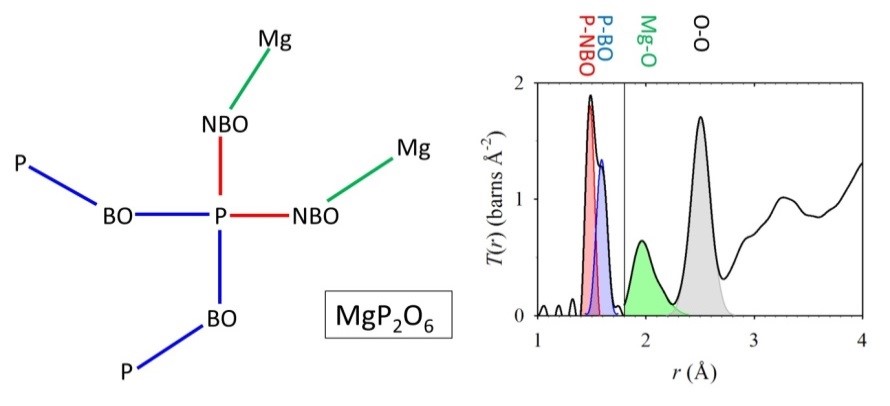
The neutron correlation function for MgP2O6 glass, and a schematic showing the atom pairs that give rise to the first few peaks (P-NBO, P-BO, Mg-O and O-O).
Stereochemical activity of Pb2+ lone pairs
O. L. G. Alderman, A. C. Hannon, D. Holland, S. Feller, G. Lehr, A. Vitale, U. Hoppe, M. von Zimmerman, and A. Watenphul, Lone-pair distribution and plumbite network formation in high lead silicate glass, 80PbO·20SiO2, Phys. Chem. Chem. Phys., 2013, 15, 8506-8519.
The high neutron energies available on GEM enable the diffraction pattern to be measured up to high maximum momentum transfer, Qmax. The high values of Qmax in turn lead to a high real-space resolution for the correlation function determined from the diffraction data. A very widely used application of this high resolution is in the study of phosphate glasses, where neutron diffraction is the only experimental method that has been able to resolve the small difference between the lengths of the bonds from phosphorus to bridging and non-bridging oxygens. In a more subtle way, the high resolution is also of advantage in the study of bond lengths that have a more complex distribution than simply being split into two distinct values, and a good example of this is given by lone pair cations in glass. On one hand, lone pair cations in glass are of current technological interest arising from their non-linear optical properties; on the other hand, lead is a very important element in glass science, and the structural role of lead in high PbO glasses has been the subject of much debate for decades.
The figure shows an EPSR simulation of the structure of a silicate glass containing 80 mol% PbO, derived from a combination of neutron diffraction data obtained on GEM, and X-ray diffraction data. The neutron diffraction data are key to the simulation, because only neutron diffraction has the real-space resolution necessary to reveal the asymmetric distribution of Pb-O bond lengths. (And the accurate coordination number information is vital, too.)
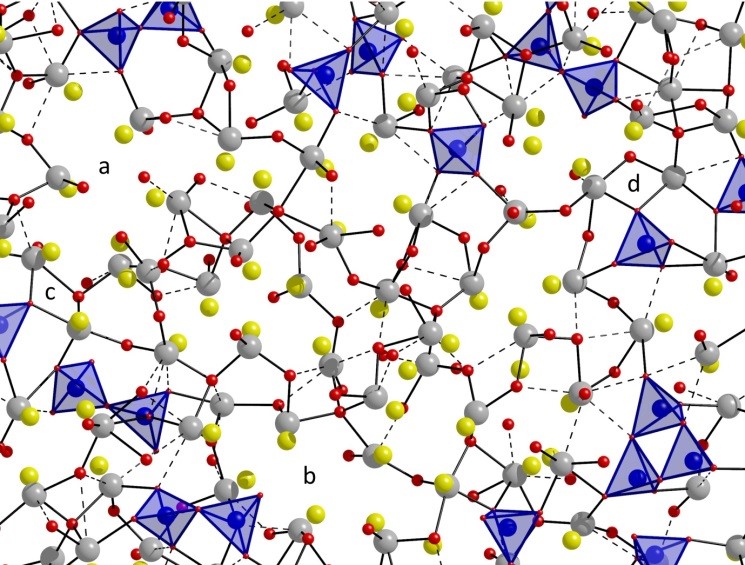
EPSR simulation of the structure of 80PbO·20SiO2 glass. Si atoms are shown within shaded blue tetrahedra; Pb atoms are shown as larger grey spheres, bonded to oxygen atoms, with an unbonded yellow sphere representing the lone-pair of electrons. The lone pairs fill voids in the glass structure, such as those labelled a and b, reminiscent of the crystal structure of a-PbO.
Mechanisms involved in the germanate anomaly
E. R. Barney, A. C. Hannon, N. Laorodphan, and D. Holland, The influence of lone-pair cations on the germanate anomaly in glass, J. Phys. Chem. C, 2011, 115, 14997-15007.
A. C. Hannon, D. Di Martino, L. F. Santos, and R. M. Almeida, Ge-O coordination in cesium germanate glasses, J. Phys. Chem. B, 2007, 111, 3342-3354.
The count rate of GEM is high compared to earlier generations of neutron diffractometer, making it possible to perform parametric studies of larger numbers of samples (or temperatures etc.) than was previously possible. For example, it has become possible to study the coordination numbers in glasses as a function of composition with significant numbers of samples, and hence compositions. This has been especially important for germanate glasses, for which there is no other experimental technique that can yield accurate and reliable coordination numbers. The composition-dependence of the Ge-O coordination number in caesium germanate glasses yields insight into the mechanisms governing the change in coordination number, and show that it is dominated by conversion between GeO4 and GeO5 units. Although Tl+ and Cs+ are both monovalent, the behaviour in thallium germanate glasses is shown to be different at high modifier contents due to the reduction in the Tl-O coordination number as the lone-pair of electrons becomes stereochemically active
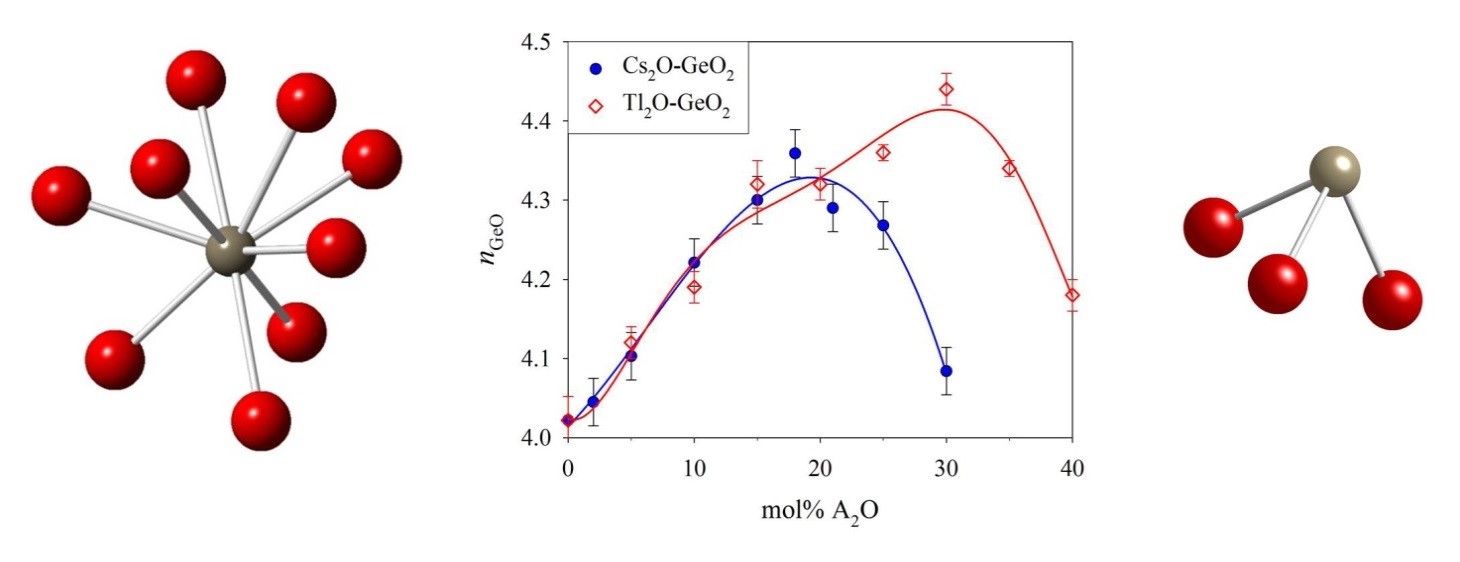
Ge-O coordination numbers for thallium and caesium germanate glasses. Also shown are typical Tl environments for low and high Tl2O contents.
PDF studies of crystalline materials
S. J. Hibble, A. M. Chippindale, E. Marelli, S. Kroeker, V. K. Michaelis, B. J. Greer, P. M. Aguiar, E. J. Bilbé, E. R. Barney, and A.C. Hannon, Local and average structure in zinc cyanide: towards an understanding of the atomistic origin of negative thermal expansion, J. Am. Chem. Soc., 2013, 135, 16478-16489.
The study of crystalline materials using the PDF method has been a great strength of the overall scientific programme on GEM. Even though the samples studied are essentially crystalline, the Disordered Materials Group has made a significant contribution to these studies, and 14% of all Disordered Materials GEM publications have been in this area. The experimental requirements for a PDF study of a polycrystalline sample are almost exactly the same as for a glass, and crystalline PDF work on GEM has benefited greatly from the instrumentation demands that arose from the Disordered Materials Group. The experience of using GEM has led to considerable blurring of the boundary between crystalline and non-crystalline studies. The method is illustrated using results for the NTE (negative thermal expansion) compound Zn(CN)2; previous studies of disordered metal cyanide crystals had not found any difference between the M-C and M-N bond lengths (M=metal atom), but fitting of a geometrical model to the detailed shape of the neutron correlation function revealed the difference in Zn–N and Zn–C bond lengths.
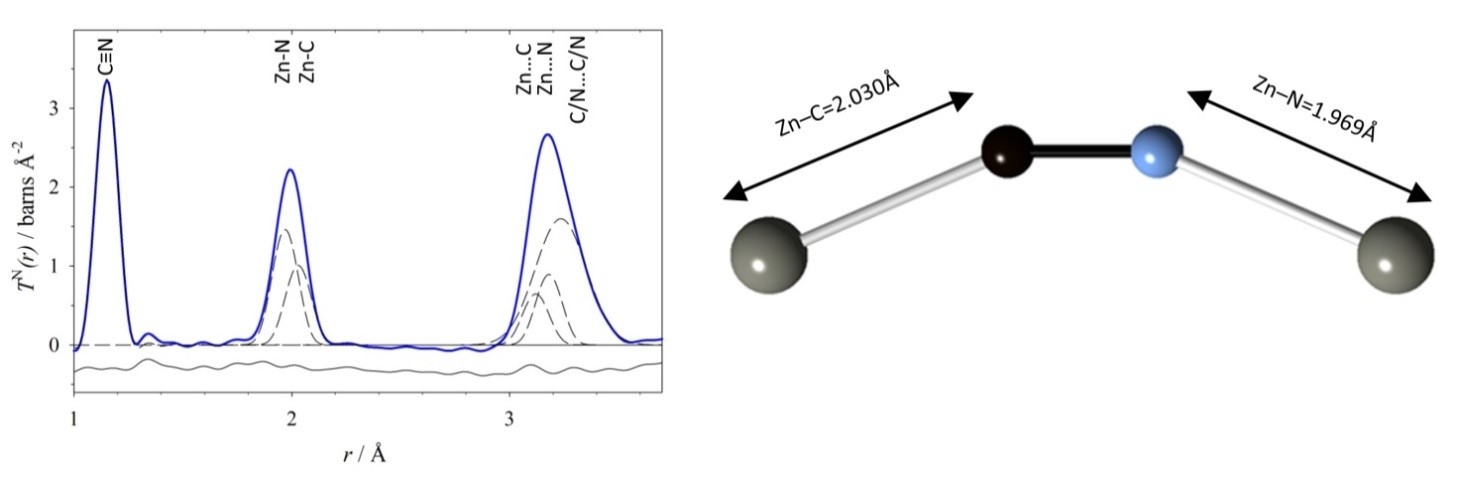
The neutron correlation function for Zn(CN)2 at 11.4 K, and a fit based on a detailed geometrical model, revealing the difference in Zn–N and Zn–C bond lengths.
Our Science Highlight articles cover the diverse research that takes place at our facility. Read all our Science Highlight articles using GEM below: