Their work, published in Nature Communications, proposes and demonstrates the first tunable beam of entangled neutrons, a fundamentally new quantum probe. Individual neutrons can be entangled in spin, trajectory and energy.
Erwin Schrödinger, originally based on systems discussed by Albert Einstein, Boris Podolsky and Nathan Rosen, first proposed the concept of quantum entanglement in the 1930s. Quantum entanglement occurs when two species are formed, and the state of one of these is fundamentally dependant on the state of the other. For example, two electrons with an overall spin of zero. One must be spin +1/2, which makes the other spin -1/2. By observing one, it is as if the communication between them occurs instantly to ensure the other has the relevant allowed state. This instant communication caused Einstein to describe the process as “spooky action at a distance", as it did not conform to his notion of local reality. Einstein believed that eventually scientists would discover a theory better than quantum mechanics and that entanglement would then be understood.
For a single neutron, the states of its spin, trajectory and energy determine its individual state. The trajectory of a particular neutron spin state can be manipulated using refraction through magnetic fields that couple with the neutron's magnetic moment. The group's experiments used a neutron spin-echo interferometer on Larmor to show that it is possible to entangle the path, spin and energy of individual neutrons on a microscopic length scale.
Their first series of measurements measured the beam intensity when the neutron spin and path were entangled for a range of spin and path phases. For the second, they entangled the energy state with the neutron spin and path states to obtain something known as a Greenberger-Horne-Zeilinger (GHZ) state. These experiments enabled them to control the entanglement characteristics of the neutron beam, and to prove them.
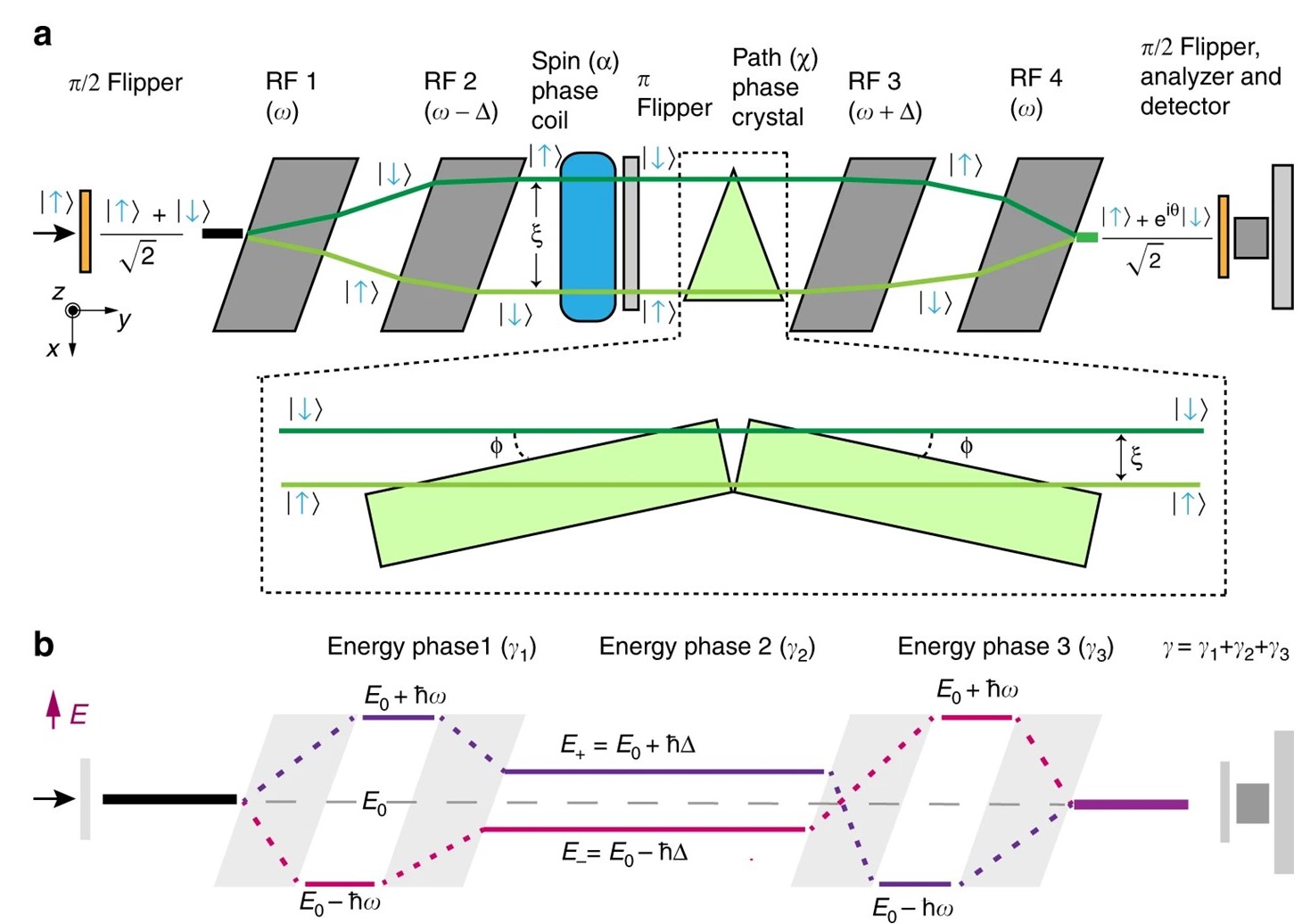
Path, Spin and Energy phase encoding using the Larmor
spin-echo setup constructed by TU-Delft
The development of this probe offers new opportunities to investigate fundamental symmetries and interactions such as the effect of gravitation on the entangled distinguishable neutron properties, such as spin. Orbital angular momentum can also be added to the list of properties to be entangled, opening the potential for advancing applications in quantum metrology and high-precision measurement.
The group aims to push the limits of quantum-enhanced metrology to explore the frontiers of fundamental physical phenomena. This could lead to novel insights into some of the most interesting materials studied today, such as frustrated magnets hosting quantum spin liquids and unconventional superconductors with strange metallic behaviour.
Further information
The full paper can be found at: https://www.nature.com/articles/s41467-020-14741-y
Read other science highlights from Larmor.